Nutriforum 2022 , Spain
You are here
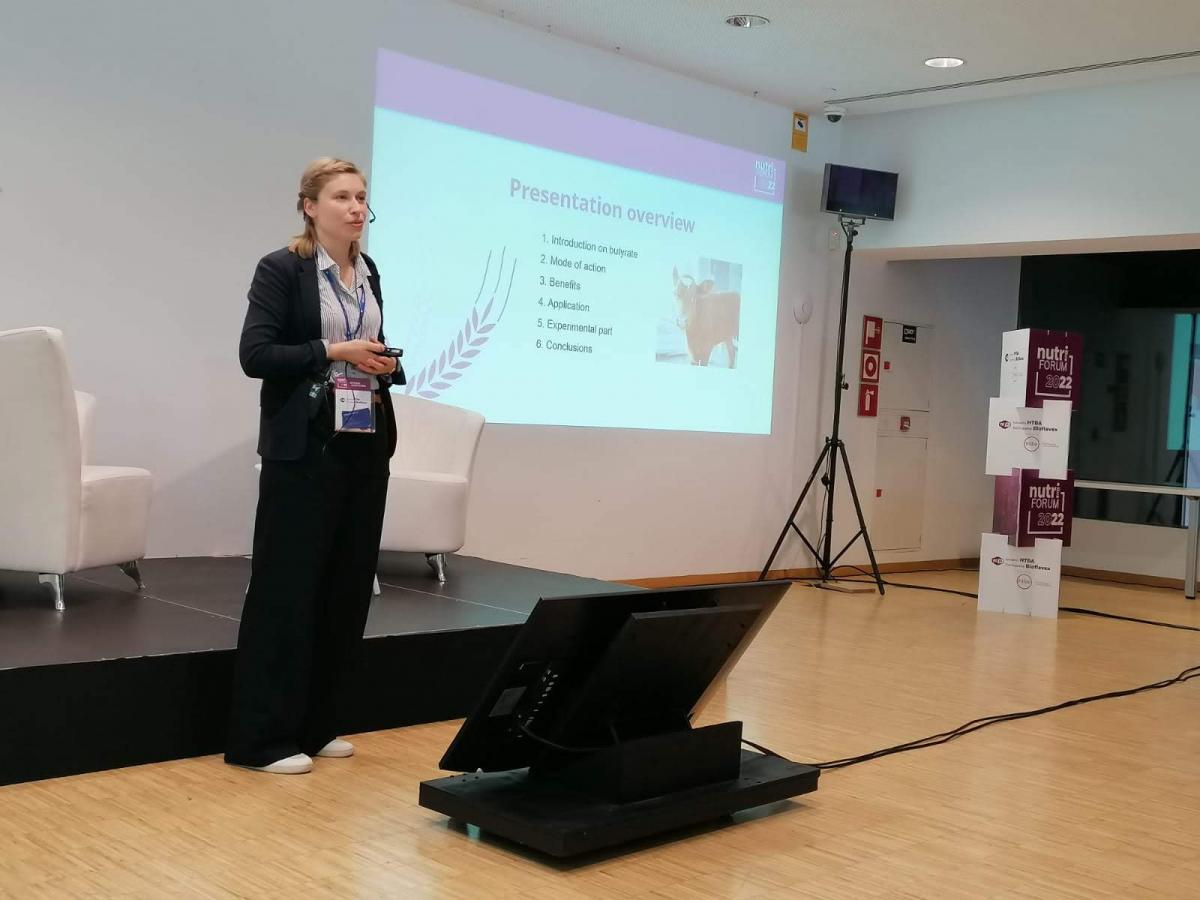
Recently, Sanluc participated in Nutriforum, the Spanish Animal Nutrition Conference in Lleida, together with his Spanish distributor Disproquima. Our R&D manager, Dr. Noémie Van Noten, was happy to give a presentation in the ruminants session about the use of coated calcium butyrate in calf milk replacers. The abstract of the presentation can be found below. If you are interested in the presentation or the topic, please feel free to contact us.
Coated calcium butyrate in calf milk replacers: mode of action, application and benefits.
Butyric acid is a short chain fatty acid and one of the major end products of bacterial carbohydrate fermentation, next to acetate and propionate. Therefore, butyric acid is naturally present in the rumen of ruminants and in the hindgut of both monogastric and ruminating species (Bergman, 1990). Moreover, bovine milk fat contains 4 to 5% butyric acid, which is mainly present on the third position of the triacylglycerols and is released by pre-gastric lipase after milk ingestion by the calf (Drackley, 2008). The presence of the butyrate anion is shown to have a whole array of potential beneficial effects on intestinal level and in the adjacent tissues (Canani et al., 2011). Since commercial milk replacers generally do not contain milk fat, but rather fat from vegetal or animal sources with a lower butyric acid content, it makes sense to supplement butyrate in the diet of young calves to ensure appropriate gastro-intestinal development (Górka et al., 2018). In practice butyrate is often supplemented in the form of its salts (mainly Ca and Na) instead of the pure acid because these salts are crystalline, less odorous and more stable.
A young calf can be classified as a pre-ruminant, since it is unable to digest solid feed due to its underdeveloped rumen at birth. The ingested liquid feed (i.e., whole milk or milk replacer) arrives directly at the abomasum via the oesophageal groove (Drackley, 2008). Although already functional, a rapid maturation of the abomasum, pancreas and small intestine is highly desirable to maintain animal growth and avoid intestinal disorders in the first weeks of life. Unprotected calcium butyrate supplemented in the milk replacer, is readily available in the abomasum and mainly stimulates the development of the proximal small intestine and pancreas, whereas the coated calcium butyrate (CCB) will gradually release the butyrate from the coating affecting also the distal small intestine. Furthermore, the development of the rumen and of its functional microbial community only begins with the ingestion of sufficient amounts of solid feed. To speed up this process, butyrate can be supplemented in the solid feed as well, since it stimulates the differentiation of the absorptive epithelium into the characteristic rumen papillae (Drackley, 2008, Mentschel et al., 2001). Again, a distinction should be made between uncoated and coated calcium butyrates. The uncoated form predominantly affects the rumen itself, as the epithelial cells will rapidly absorb the dissolved butyrate for internal use. Contrarily, the coated form affects not only the rumen, but is also expected to partly bypass the forestomach and the abomasum in young calves, hence exerting effects in the small intestine (Górka et al., 2018).
The stimulatory effects of butyrate on gastro-intestinal cell development and function might be of direct origin since butyrate is the preferred energy source for rumen epithelial cells and colonocytes. However, butyrate has also been shown to increase cell proliferation, decrease apoptosis of healthy cells and regulate epithelial cell function in general. The latter observations suggest an indirect mechanism in which butyrate acts as a signal metabolite (Guilloteau et al., 2010; Górka et al., 2018, Mentschel et al., 2001). To date, four G protein-coupled receptors (GPCRs) have been identified which are activated by butyrate (FFAR2, FFAR3, GPR109a and OR51E1). These receptors are not only present on enteroendocrine cells, but also on immune cells and adipocytes. Through these receptors butyrate might modulate various physiological processes, such as the secretion of digestive enzymes, secretion of hormones that regulate appetite and energy homeostasis, or immune reactions (Stilling et al., 2016; Guilloteau et al., 2010). Apart from its interaction with receptors, butyrate influences cellular signalling pathways by acting as a histone deacetylase (HDAC) inhibitor. HDACs are a family of enzymes that catalyse the removal of acetyl groups from lysine residues on histones, which allows the more tightly wrapping of DNA around these histones and hence DNA silencing. Butyrate exerts many of its anticancer activities, as well as part of its anti-inflammatory effects through HDAC inhibition. Additionally, this regulation of gene expression might also contribute to butyrate’s beneficial effects on gut development and function (Liu et al., 2018, Stilling et al., 2016). Finally, butyrate is known for its microbiota modulating action and more specifically for the inhibition of colonisation of pathogens like Salmonella spp and Clostridium perfringens (Guilloteau et al., 2010, Van Immerseel et al., 2004). The effect on microbiota might be explained by a direct bactericidal activity towards gram negative bacteria, as well as an indirect effect caused by general improvement of the intestinal barrier function, digestion and absorption (Moquet et al., 2016).
Taken together, the beneficial effects of butyrate supplementation result in healthier and better performing animals. This is also documented by several trials performed with CCB in calves at doses ranging between 1.25-5.2 kg/ton milk powder. The effect on intestinal morphology was illustrated in a trial using 50 veal calves with an average age of 94 days. The animals were divided over 5 dietary treatments (n=10) for 12 days during which they received a conventional milk replacer, a modified milk replacer with 8% of soy protein (MMR) or a MMR supplemented with 1.28, 2.56 or 5.12 kg/ton CCB. Feeding a milk replacer with plant protein significantly reduced the villus height in the jejunum as compared to conventional milk replacer (1131 vs 1305 µm, respectively). Supplementation of CCB to the MMR resulted in a dose-dependent increase in jejunal villus height with values of 1241, 1380 and 1511 µm for the doses of 1.28, 2.56 or 5.12 kg/ton CCB, respectively. In two independent performance trials with younger calves, it was demonstrated that the average daily weight gain can be improved with approximately 5% and the feed conversion ratio with approx. 3% when adding CCB to the milk replacer.
In conclusion, the application of coated calcium butyrate in calf milk replacers or in solid feed has proven to be beneficial for the early development of a functional gastro-intestinal tract resulting in high performing and healthy calves.
References
- Bergman, E. N. (1990). Energy contributions of volatile fatty acids from the gastrointestinal tract in various species. Physiological reviews, 70(2), 567-590.
- Canani, R. B., Di Costanzo, M., Leone, L., Pedata, M., Meli, R., & Calignano, A. (2011). Potential beneficial effects of butyrate in intestinal and extraintestinal diseases. World journal of gastroenterology: WJG, 17(12), 1519.
- Drackley, J. K. (2008). Calf nutrition from birth to breeding. Veterinary clinics of North America: Food animal practice, 24(1), 55-86.
- Guilloteau, P., Martin, L., Eeckhaut, V., Ducatelle, R., Zabielski, R., & Van Immerseel, F. (2010). From the gut to the peripheral tissues: the multiple effects of butyrate. Nutrition research reviews, 23(2), 366-384.
- Górka, P., Kowalski, Z. M., Zabielski, R., & Guilloteau, P. (2018). Invited review: Use of butyrate to promote gastrointestinal tract development in calves. Journal of dairy science, 101(6), 4785-4800.
- Liu, H., Wang, J., He, T., Becker, S., Zhang, G., Li, D., & Ma, X. (2018). Butyrate: a double-edged sword for health?. Advances in Nutrition, 9(1), 21-29.
- Mentschel, J., Leiser, R., Mülling, C., Pfarrer, C., & Claus, R. (2001). Butyric acid stimulates rumen mucosa development in the calf mainly by a reduction of apoptosis. Archives of Animal Nutrition, 55(2), 85-102.
- Moquet, P. C. A., Onrust, L., Van Immerseel, F., Ducatelle, R., Hendriks, W. H., & Kwakkel, R. P. (2016). Importance of release location on the mode of action of butyrate derivatives in the avian gastrointestinal tract. World's Poultry Science Journal, 72(1), 61-80.
- Stilling, R. M., van de Wouw, M., Clarke, G., Stanton, C., Dinan, T. G., & Cryan, J. F. (2016). The neuropharmacology of butyrate: the bread and butter of the microbiota-gut-brain axis?. Neurochemistry international, 99, 110-132.
- Van Immerseel, F., Fievez, V., De Buck, J., Pasmans, F., Martel, A., Haesebrouck, F., & Ducatelle, R. (2004). Microencapsulated short-chain fatty acids in feed modify colonization and invasion early after infection with Salmonella enteritidis in young chickens. Poultry Science, 83(1), 69-74.